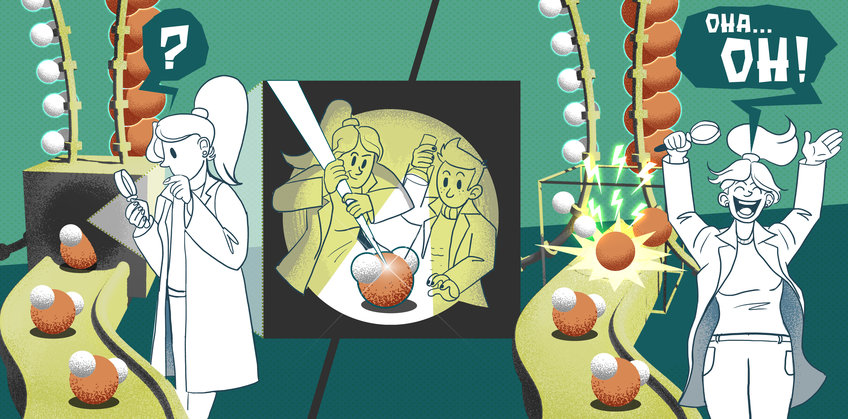
How to split water
It's holiday time again. Traffic is heavy on the roads, and many people are heading south. But there is no sign of pollution in the air. It’s the year 2050, when fuel-cell cars are an everyday reality and the energy for cars is generated from molecular hydrogen. Hydrogen charging stations have replaced the network of fossil fuel stations.
This is only one of many possible visions for the future. The question of how enough molecular hydrogen could be obtained for this purpose also remains unanswered. The simplest source of H2 is water, from which pure hydrogen can be obtained by electrochemical water splitting. However, though the process of water splitting may sound simple at first, a microscopic understanding of it is still lacking. However, this understanding is essential in order for the most efficient electrochemical energy conversion systems possible to be realised in the near future.
Water splitting – a black box
Everybody has seen the electrolysis experiment at school. Two electrodes are immersed in water containing some acid. When a voltage is applied between the electrodes, small bubbles are generated. Oxygen, i.e. O2, forms at the positive pole while H2 forms at the negative pole. Sometimes the reverse process can also be seen, for example whereby a fuel cell produces water from H2 and O2 generating electricity that can be used to drive a motor.
At school, however, it is not explained how exactly water is converted at the electrodes during electrolysis or in the fuel cell – not because it is too complicated but because this process is not yet understood in detail.
With our research, we aim to understand how these two closely related processes occur at molecular length scales and how they proceed over time. We tackle questions such as, for example, what effect does the roughness of the surface of the electrode have on the efficiency of the reaction? How exactly does the splitting process or conversely the combustion proceed from an electrochemical perspective? Which intermediates are formed during the reaction and how quickly do they dissipate? Finding answers to questions like these makes a significant contribution towards developing more efficient electrodes tailored to the reactions in question.
How to shed light on water in the dark
But how it is possible to study water splitting at the molecular length scale under reaction conditions? Also, how can you retain chemical selectivity – i.e. how to detect different intermediates that are formed during the process?
In our research, we use Raman spectroscopy for this purpose. When excited by laser light, the various atoms that make up molecules can swing against each other like balls connected with springs. A different amount of energy, which is provided by the laser, is required for the vibration depending on the type of atom. The backward-scattered light from the sample then has a molecule-specific fingerprint, the Raman spectrum.
Oh, OH!
To study electrochemical reactions, we shine laser light onto the surface of a potential-controlled electrode and record a Raman spectrum every half a second. The fingerprints of the molecules involved in the process briefly appear and disappear again in the spectra. With the help of computer simulations, the fingerprints can be assigned to reaction intermediates.
An unexpected finding of our measurements is that after applying a voltage to a gold electrode covered with oxygen atoms – that is, an oxidised gold electrode – in acidic water, couples of hydrogen and oxygen atoms, i.e. OH groups, are stable on the gold atoms for a few seconds. The formation of these kinds of OH groups has been predicted using theory for a long time but could not be confirmed by means of experiments until now. Our experimental evidence now allows us to narrow down the number of possible reaction pathways, paving the way for the design of more efficient electrode surfaces. Our results also suggest that OH groups may be an important intermediate in the reverse process, i.e. in water splitting.
Cutting-edge research with high potential
These measurements however do not yet answer the question of how the atoms of an electrode surface should ideally be arranged to optimise the efficiency of water splitting. To gain a more detailed insight we have used tip-enhanced Raman spectroscopy, where the apex of a fine metal wire only a few hundred atoms in thickness is moved closely above the surface. The tip scans across the surface – similar to how a finger reads Braille, only much more sensitive. Furthermore, the tip acts as an antenna and amplifies the Raman signal scattered from beneath the apex so that the fingerprint of a few molecules can be recorded with nanometer spatial resolution.
In our experiments, we placed a tip of this kind in aqueous electrolyte and used it to study the reaction on the electrode surface live at the molecular level for the first time. The Raman nano-images show that the process of water splitting is particularly efficient at nano-sized protrusions, and that atomic differences in surface structure lead to distinct reaction intermediates which are chemically identified – important information to know when designing optimised electrodes.
Interesting not only for water
So far, we have only carried out our water/hydrogen research on model electrodes. Now, however, our newly-developed investigation methods are ready to be applied to other electrode materials and also to provide insights into other industrially relevant chemical processes, such as for example electrosynthesis reactions – in addition to making fundamental contributions to the design of more efficient energy conversion devices, which may be a small but important step towards hydrogen-powered cars.