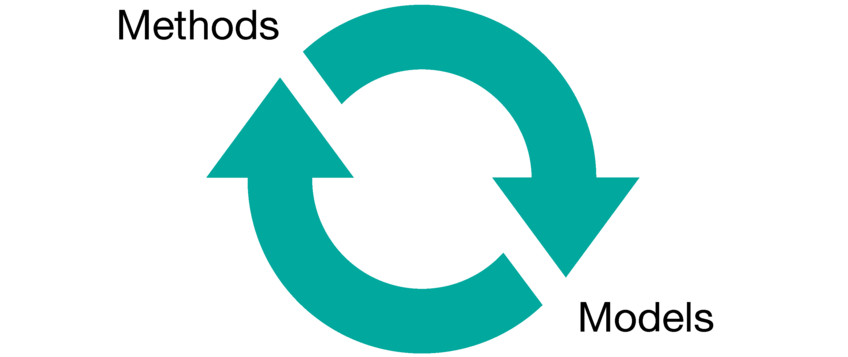
Methods | Dept. Bonn
In our department we combine different methods to obtain a better understanding of the microscopic mechanisms related to our three main research themes: water, biomolecules and charge-carrier dynamics. Based on our measurements we proposed models to describe the systems that are then tested with further experiments.
Infrared and two-dimensional Infrared Spectroscopy
Infrared absorption spectra sensitively report on molecular vibrations and allow for detecting the impact of the environment on such molecular vibrations. In ultrafast infrared pump-probe spectroscopy and two-dimensional infrared spectroscopy (2D-IR), a short and intense infrared laser pulse is used to ‘label’ a specific vibration via vibrational excitation. Monitoring the temporal and angular evolution of this excitation provides information on energy transport or molecular motion in real-time. The effect of an excited vibration on other molecular vibrations, which can be assessed in a 2D-IR experiment, provides direct evidence for molecular interaction.
Raman
Raman scattering is the inelastic scattering of light from molecules or solid state materials. Raman spectroscopy reports on the vibrational (or rotational, or phonon) modes of the system under investigation and provides chemical information about molecules and their interaction with their environment. A monochromatic light (usually from a CW laser in the visible, near IR or UV) is used for excitation, and the Raman shift, i.e. the energy difference between the incident and scattered radiation, reports on the characteristic vibrational (or rotational, or phonon) modes of the system, according to the Raman selection rules. Raman spectra reveal similar, complementary information to IR spectra.
Surface & Tip Enhanced Raman Spectroscopy
Surface- and tip-enhanced Raman scattering spectroscopies are based on plasmonic near-field effects that can enhance Raman scattering by up to seven orders of magnitude, thus enabling Raman vibrational spectroscopy of few molecules as present in surface adlayers, or even of single scatterers in special cases. Combined with an electrochemistry platform, SERS or TERS studies at electrified solid/liquid interfaces are feasible.
Coherent Anti-Stokes Raman Spectroscopy
One way to enhance the inherently weak Raman scattering from molecules is to enforce coherent scattering with short, intense laser pulses. In coherent anti-Stokes Raman spectroscopy (CARS), three photons interact with the sample molecules in a way that a fourth photon at the anti-Stokes energy is scattered. Through the coherent signal emission and nonlinear nature of the wave-mixing approach, the CARS signal is enhanced by three to five orders of magnitude compared to conventional Raman scattering, rendering CARS a valuable novel tool for in situ quantitative monitoring of dynamic molecular processes at the microscale. Commonly, CARS is employed in the field of bio(medical) imaging and has only recently been introduced to the field of materials science.
THz spectroscopy
Covering the energy range from 1-100 meV, terahertz spectroscopy is a powerful tool to investigate the low energy excitations and charge carriers (e.g. free carriers, polarons, and excitons.) in inorganic/organic solids and their nanostructures. Employing optical pump (pulsed) THz probe spectroscopy, the dynamics of charge carrier generation (e.g. hot carrier cooling and exciton formation), transport through the photo-active materials (e.g charge scattering time and mobility), and charge transfer across interfaces can be directly studied with sub-ps time resolution.
Microwave and Terahertz Dielectric Spectroscopy
Dielectric spectroscopy measures the response of a sample to an externally applied, oscillating electric field. At microwave to Terahertz frequencies, the polarization of liquids stems from the rotation of dipoles and/or short-ranged fluctuations of ions. As such, dielectric spectroscopy allows quantifying molecular dipoles and their rotational mobility. Formation of molecular or ionic aggregates (like e.g. ion-pairs) with large electric dipoles is detected and used to quantify intermolecular interactions and solvation.
Two-dimensional Terahertz-InfraRed-Visible (2D TIRV) spectroscopy
Two-dimensional Terahertz-InfraRed-Visible (2D TIRV) spectroscopy measures correlations (couplings) between the low-frequency vibrations (in the terahertz frequency range) and high-frequency vibrations (in the mid-infrared frequency range). This coherent nonlinear spectroscopy technique uses mixing of the terahertz (THz), infrared (IR) and visible light pulses in a sample to generate new signal wave. The emitted signal is measured for different THz and IR frequencies. The signal is enhanced when the THz and IR fields are in resonance with low- and high-frequency molecular vibrations, respectively, and when these vibrations are coupled. Information about the coupled vibrations provides new insight into the nature of the low-frequency modes, which are intramolecular motions of large molecular segments or intermolecular motions of molecules relative to each other.
Surface sensitive non-linear optical techniques
Sum frequency and second harmonic generation (SFG and SHG) have become reliable tools that provide surface-specific information. In an SFG experiment, an infrared (IR) and a visible (VIS) laser pulse are overlapped in time and space at a surface, leading to the generation of photons at the sum-frequency of the IR and VIS frequencies. Vibrational information is obtained when the IR frequency is tuned into resonance with a vibrational mode. SHG is the degenerate case of SFG when only one laser beam is used and photons at twice the frequency of the incident laser are generated. Information on an electronic transition can be obtained from SHG when either the frequency of the incident laser or the emitted frequency-doubled photons are resonant with an electronic transition. SFG and SHG owe their surface specificity to the selection rule that symmetry must be broken, which evidently applies at the phase boundary between two media.
These techniques can be used in different modes and can provide information on orientation, kinetics, and dynamics and can be applied not just for the study of planar interfaces, but also to the surface of nanoparticles.
X-ray Photoelectron Spectroscopy (XPS)
XPS is a surface technique with high surface sensitivity (~10 nm) and low elemental detection limit (~0.1 %). For an XPS measurement, the sample is placed in ultra-high vacuum and irradiated with X-rays with given energy; this results in the ionization of the atoms and emission of the core-level electrons. Analyzing the emitted photoelectrons provide information of the surface species including elemental composition, chemical state and molecular packing.
Langmuir Trough
Surfactants at the air/water interface form Langmuir monolayers that can be compressed by closing the lateral barrier of the trough to a given surface pressure or at a given area per molecule. The trough is usually equipped with a vertical dipper than enable Langmuir-Blodgett or Langmuir-Schaefer type of deposition to transfer the compressed monolayer onto solid substrates and it also allows the recording of surface-area isotherms.
Brewster Angle Microscopy
Brewster’s angle is a special incidence angle at which no p-polarized light is reflected from the air/water interface. Thus, at this incidence angle the collected image in p-polarization is completely dark. By spreading surfactant molecules at the air/water interface the Fresnel coefficient for p-polarized light will change and thus the reflected intensity will be different from zero. The images thus taken by the camera will show the formation of the monolayer (grey on top of dark background) and provide information on its morphology.
Ellipsometry
Ellipsometry is a linear optical technique that measures the change in light polarization upon interaction with a sample. When it is performed at a single wavelength it allows for the determination of the thickness of the film once its refractive index is known.
Molecular Dynamics Simulation
MD simulation is a technique to monitor the molecular motion in time. By combining MD simulation with the vibrational spectra modeling, we are able to compute the vibrational spectra. We are currently using ab initio molecular dynamics simulation technique as well as force field molecular dynamics simulation technique.
Microscopy
Microscopy both in confocal and wide-field geometry is available with the option to use different nonlinear optical modalities of light-matter interaction such as stimulated Raman scattering.