
Welcome to the cell!
Project “MaxSynBio” produces artificial cells
"We are now in the membrane that protects our cell, ladies and gentleman," says the cap-clad tour guide. A small, spaceship-like vehicle with a large glass dome moves through an orange-blue, biological-looking environment. The first episode of the series "Once Upon a Time… Life", which was produced in 1986, shows the processes that take place in cells. What quickly becomes clear in the 25-minute animated film: The processes in cells are complex! Golgi apparatus, mitochondria, lysosomes and many other parts work together to keep the cell alive, to supply it with energy or even to ward off diseases such as viruses.
"We wanted to take cells like this as an example and artificially reproduce individual functions," says Katharina Landfester, director at the Max Planck Institute for Polymer Research. She heads a subproject of the cross-institute project "MaxSynBio." The goal of this subproject: to generate artificial miniature cells. "Only by rebuilding cells ourselves step by step, we can understand how real cells work," says Landfester. She adds: "In the end, we'll have a better understanding of how life works at its fundamental level."
Cells from the toolbox
To create artificial cells, the scientists around Landfester basically need two components: A shell, called a "compartment," which protects the contents, and the microscopic contents. Together, the shell and the contents determine which function the artificially produced cell can perform.
The goal of the "MaxSynBio" subproject at the Max Planck Institute for Polymer Research is to create a toolbox filled with parts and methods that can be used to produce such artificial cells from scratch. In this way, cells that perform different tasks - such as mimicking biological functions - can be assembled by "simply" putting together the right parts.
This principle can be used, for example, to create artificial cells that can synthesize special molecules. For this, the right catalysts - i.e., the right "content" - must be built into the right synthetic vesicle - i.e., compartment. Or if cells are needed that can form tissue-like structures, this can be achieved by incorporating light-responsive components into the synthetic vesicles that attach to each other when exposed to light, forming a mesh of interconnected vesicles.
A house in miniature
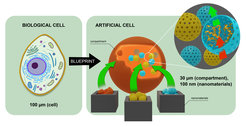
An important step in the project was the fabrication of compartments that surround and protect the interior of the cells: These miniature containers - the vesicles - cannot simply be made by hand, because with a size of only 20-30 micrometers - millionths of a meter - they are far too small for that. Sophisticated manufacturing methods are needed to produce these shells. And that's not all: When manufacturing the shells, all the components that one wants to have inside the artificial cell must also be integrated at the same time!
To make the shells, the team of scientists work with a special type of polymer: a long-chain molecule called a block copolymer. This materials can entangle with neighboring block copolymers in the presence of water to create a stable shell.
The block copolymers themselves are made by chemically joining two "blocks": A "water-avoiding" polymer chain is joined to a "water-seeking" chain. Once such a material comes into contact with water, the water-avoiding blocks attempt to orient themselves so that they are protected from the water. This creates a membrane with an essentially "dry" layer surrounded by the protective "water-seeking" block, which is in direct contact with water. By using the right materials for each block and adjusting their length, it is thus possible to create polymer vesicles by placing these materials in contact with water.
In their research, the scientists went a step further and developed a variety of such block copolymers for their molecular toolbox to be flexible depending on the application: for example, some of them can only self-assemble when irradiated with light, while others consist of special water-seeking blocks that self-assemble much more efficiently than other materials.
Function follows form
In parallel with research into the possibilities of producing vesicles with special properties, another part of the project is dealing with the production of the active components located inside the shells. For this purpose, as in the production of the vesicles themselves, chemical methods are used to produce small nanoparticles. These resemble a sponge or a hollow container and can be loaded with chemicals, such as drugs or catalysts. These very small particles are typically 100 nanometers in diameter - or 100 billionths of a meter. But the tiny particles are huge when it comes to their function.
In Katharina Landfester's department, nanoparticles and nanocapsules are produced by first creating very small droplets dispersed in either oil or water. Reactive chemicals, called monomers, present in the droplets can start a polymerization: they combine with each other when exposed to a trigger - called an "initiator”. Because this polymerization is confined to the tiny volume of the droplet, the process results in solid nanoparticles or nanocapsules with a shell surrounding a liquid core. These can be used as tiny packaging for transporting functional materials such as enzymes. One advantage of this approach is that the load - for example, the enzymes - are protected by the solid nanoparticle material, allowing them to work more efficiently than if they were used freely in solution.
If special monomers are used, the resulting nanoparticles can also have tunable chemical properties. For example, it can be ensured that a reaction is only triggered when the nanoparticle is illuminated with light.
Energy

In the future, lost cell function could be restored with artificial cells.
But what can be achieved with such a ready-made test system? What can it be used for? "We think that in the future such systems can be used in the medical field," Landfester says.
In the human body, for example, the molecule 'NAD' plays a major role in supplying energy to cells: Glucose, or sugar, must be converted into ATP in cells. In a sense, ATP is the energy supplier of the cells. In this conversion process from glucose to ATP, NAD acts as an electron carrier. In this process, NAD is converted into NADH - a similar, yet different substance. NAD is therefore "consumed”.
If new NAD is needed, for example because the body wants to convert glucose to ATP again, the body produces the molecule in a targeted manner at the places and times where it is needed.
"We now wanted to take a different approach and recover NAD from NADH - whenever we wanted it," Landfester explains. To do this, the researchers created a completely synthetic system that can produce NAD from NADH - in other words, "recycle" NADH. The process is triggered whenever the system is irradiated with light and can thus be controlled externally.
The first test system, which is already functional in the laboratory set-up, clearly demonstrates that the combination of synthetic components can help to replicate biological processes in the future and to control them by external triggers, like for example light.
"A variety of applications for this technology are conceivable," Landfester explains. "For example, it could be used as a new form of therapy in the future: One could use miniature cells to stabilize processes that are out of balance in the body and thus support natural cell function."
It will probably take many more years of research and development until this point is reached. But with the "MaxSynBio" project, new tools are already being developed today that will make it possible in the future to create complex cell-like structures that can be used as tiny biological machines.